A meta-analysis of exposure to particulate matter and adverse birth outcomes
Article information
Abstract
Objectives
The objective of this study was to conduct a systematic review to provide summarized evidence on the association between maternal exposure to particulate air pollution and birth weight (BW) and preterm birth (PTB) after taking into consideration the potential confounding effect of maternal smoking.
Methods
We systematically searched all published cohort and case-control studies examining BW and PTB association with particulate matter (PM, less than or equal to 2.5μm and 10.0 μm in diameter, PM2.5 and PM10, respectively) from PubMed and Web of Science, from January 1980 to April 2015. We extracted coefficients for continuous BW and odds ratio (OR) for PTB from each individual study, and meta-analysis was used to combine the coefficient and OR of individual studies. The methodological quality of individual study was assessed using a standard protocol proposed by Downs and Black. Forty-four studies met the inclusion criteria.
Results
In random effects meta-analyses, BW as a continuous outcome was negativelyassociated with 10 μg/m3 increase in PM10 (-10.31 g; 95% confidence interval [CI], -13.57 to -3.13 g; I-squared=0%, p=0.947) and PM2.5 (-22.17 g; 95% CI, -37.93 to -6.41 g; I-squared=92.3%, p <0.001) exposure during entire pregnancy, adjusted for maternal smoking. A significantly increased risk of PTB per 10 μg/m3 increase in PM10 (OR, 1.23; 95% CI, 1.04 to 1.41; I-squared=0%, p =0.977) and PM2.5 (OR, 1.14; 95% CI, 1.06 to 1.22; I-squared=92.5%, p <0.001) exposure during entire pregnancy was observed. Effect size of change in BW per 10 μg/m3 increase in PM tended to report stronger associations after adjustment for maternal smoking.
Conclusions
While this systematic review supports an adverse impact of maternal exposure to particulate air pollution on birth outcomes, variation in effects by exposure period and sources of heterogeneity between studies should be further explored.
Introduction
Maternal exposure to air pollution during pregnancy is associated with increased risk of adverse birth outcomes such as low birth weight (BW) and preterm delivery [1-3]. These adverse outcomes have been suggested to be associated with increased neonatal morbidity and mortality as well as possible developmental problems in childhood and risk of various diseases including depression or other psychiatric conditions in adulthood [4-6]. More than 20 million infants worldwide, representing 15.5% of all births, are born with low BW ( < 2500 g); prevalence in developing countries (16.5%) is more than double the level in the developed regions (7%) [7]. Preterm birth (PTB) is one of the reasons for low BW and is also regarded as an indirect cause of neonatal death. Risk factors of PTB include younger maternal age, smoking, and poor housing, along with medical factors such as induction, premature rupture of membranes, infection, multiple pregnancy, intrauterine death, fetal and uterine abnormalities, and chorioamnionitis [8].
Several case-control and cohort studies have revealed a positive association between maternal exposure to ambient air pollution and a range of adverse pregnancy outcomes [9-11]; however, there are notable inconsistencies among the findings of these studies [12-15]. Additionally, several reviews have been published in the last decades [16-18], but they have generally been based on a small number of studies, and only two have provided pooled estimates of effect size (ES) for BW and PTB [1,3]. These reviews provided the pooled effect estimates of -16.77 g (95% confidence interval [CI], -20.23 to -13.31, 1980 to 2011) per 20 μg/m3 increase in particulate matter (PM) less than or equal to 10 μm in diameter (PM10) for change in BW [3] and the combined odds ratio (OR) of 1.02 (95% CI, 0.99 to 1.04, till July 2009) per 10 μg/m3 increase in PM10 for PTB during entire pregnancy [1]. However, in several individual studies, we could not rule out the consequences of specific biases which may arise from differences in the methodological quality and the study settings such as the demographic characteristics of the study population, exposure periods (month, trimester, and other periods), study periods, and the degree of confounding [13,14,16,19]. In addition, some individual studies were criticized for inadequate control of smoking [20,21].
This study aimed to conduct a systematic review to provide summarized evidence of the associations between PM (less than or equal to 2.5 μm in diameter. PM2.5 and PM10) and BW and PTB, by providing summary estimates of effect by gestational period and taking into consideration the potential confounding effect of maternal smoking.
Materials and Methods
Inclusion Criteria and Search Strategy
We performed a systematic literature search in PubMed (www.ncbi.nlm.nih.gov/pubmed) and Web of Science (www.isiknowledge.com) to identify all published case-control and cohort studies (January 1980 and April 2015) that evaluated the association between PM and BW and preterm delivery, by using the search strategy described in Figure 1. Additional publications were identified based on references citied within the published articles and citation tract. Search terms included “air pollution” OR “particulate matter” OR “PM” OR “PM10” OR “PM2.5” OR “TSP” AND “low BW” OR “BW” OR “ preterm birth” OR “PTD” OR “preterm delivery”OR intra-uterine growth-retardation” OR “IUGR”. Initial screening of the studies was based on titles/abstracts. In general, studies that were not related to “air pollution” and “birth outcomes” were excluded. Furthermore, daily time series studies, case reports, case series, and studies available only in abstract form were excluded. Articles that passed the initial screening were reviewed independently by two authors to evaluate whether or not they should be considered for full review. A disagreement between the two authors was resolved by consensus. We carefully checked the references of each publication and uploaded all relevant studies into Ref Works (ProQuest, Kanagawa, Japan), and duplicate records were removed.
Data Extraction
After full review, data extraction from relevant studies was performed independently by two investigators, using standard template. The template included information on study design, location, dates of data collection, data sources, sample size, descriptive information on study subject characteristics, outcome frequency, distribution of exposure, method of exposure characterization, statistical analysis methods, ES estimates, and covariates examined jointly with air pollution. We extracted the smoking adjusted and smoking unadjusted effect measures separately from the original studies. As studies did not all include or adjusted for the same gaseous pollutants, single pollutant models fully adjusted for other covariates were used. Data were analyzed using Excel 2007 (Microsoft, Redmond, WA, USA) and Stata version 13.0 (Stata Corp., College Station, TX, USA).
In meta-analyses, we selected the results that were based on the larger number of observations for the same outcome, pollutant, and population. We selected the study of Morello-Frosch et al. [22] over those from Parker et al. [23] and Basu et al.[24] for the results of PM2.5 because the former study covered a large number of observations. Likewise, the results from Suh et al. [25] (vs. Suh et al. [11]) for PM10 and from Gray et al. [26] (vs. Gray et al. [27]) for PM2.5 were selected for meta-analysis. Instead of including the study that reported sensitivity analyses [28], we included study that reported the primary results [10]. Two studies of Jedrychowski et al. [29,30] that reported results for PM2.5 and BW for different gestational periods were included, whereas one other study only reported results based on categorical exposures [31].
Assessment of Quality
To assess the methodological quality of articles that were included for the quality comparison across studies, we used the checklist developed by Downs and Black [32]. The checklists are applicable for any analytical study related to health care intervention. The checklist consists of 27 items distributed in five subscales, including reporting (10 items), external validity (3 items), bias (7 items), confounding (6 items), and power (1 item). Answers are generally scored 0 or 1, with the exception of one item in the reporting subscale (score range, 0 to 2) and another item on power (score range, 0 to 5). According to this checklist, a study can get maximum score of 32. Two reviewers had independently reviewed each study included in the analysis, and there were no substantial difference between the judgments of these two reviewers regarding the quality of individual studies. The quality of a study was determined through comparing its score with the median score calculated for all studies included in meta-analysis. A relatively high quality study referred to a score greater than or equal to the median; otherwise, the study was considered as relatively low quality.
Statistical Analysis
In order to facilitate comparisons of ES from different studies, all risk estimates were converted to a common exposure unit of 10 μg/m3 increase in PM10 or PM2.5. Effect estimates were grouped by gestational period (trimester-specific and whole pregnancy). Most effect estimates relating to PTB were expressed as adjusted OR, however, when relative risks were reported, we converted these to ORs using the approximation approach described by Zhang and Yu [33]. The systematic review was conducted in accordance with Meta-analysis of Observational Studies in Epidemiology guidelines [34]. The pooled effect measures were estimated based on a random effect model [35], quantifying heterogeneity among estimates from primary studies suing the I-squared statistic (25%, 50%, and 75% were used as rules of thumb for low, moderate, and high heterogeneity) [36]. A potential publication bias across the included studies was examined using contour-enhanced funnel plot and Egger’s test.
We performed sensitivity analyses and subgroup analyses to evaluate potential sources of heterogeneity across the included studies. Sensitivity analyses were performed by removing each study one-by-one. Subgroup analyses were based on the possible confounding effects of smoking and the methodological quality of the studies (high or low).
Results
Study Characteristics
Table 1 summarizes the main characteristics of individual studies. A total of 44 articles that met the inclusion criteria were included in this review. Most studies employed a retrospective cohort design using administrative birth data, while four were case-control studies. Individual studies were based on as few as 235 and as many as 3303834 births. More than half of the studies (n = 25) were from North America, followed by Asia (n = 7), Europe (n = 6), Australia (n = 4), and South America (n = 2). The majority of studies (n = 32) used central monitoring data for the assessment of exposure. Three studies employed personal monitoring, while nine modeled exposures using pollutant dispersion and/or land-use patterns. Almost all studies adjusted for mother’s age, education, gestation, parity, gender, and/or socioeconomic status as a covariate and many of them also adjusted for race and season, but 14 did not directly adjust for smoking. Most of these studies stated that the smoking-unadjusted effect estimates obtained from their study was reasonably unconfounded by smoking [20,21,37].
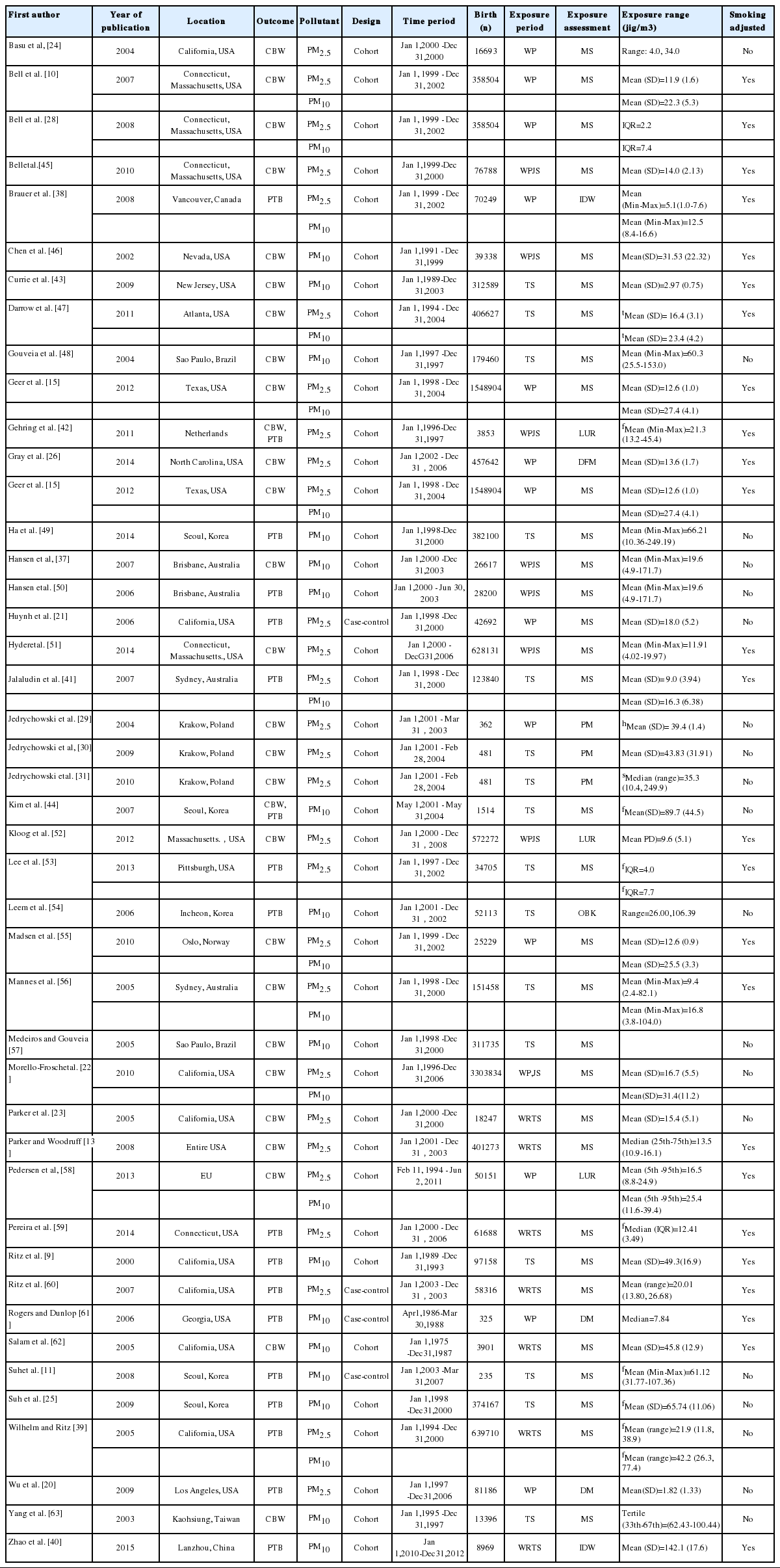
Characteristics of primary studies related to exposure to particulate matter and BW and preterm birth
For outcome of BW, there were seven studies that examined PM10 exposure and 11 studies using PM2.5, with nine studies providing estimates for both pollutants. Similarly, for PTB, there were five studies relying on PM2.5, nine studies on PM10, and four studies on both pollutants. For PTB studies, the reported mean exposure levels ranged from 5.1 μg/m3 [38] to 21.91 μg/m3 [39] for PM2.5 and 12.5 μg/m3 [38] to 142.1 μg/m3 [40] for PM10. Likewise, for BW, the measured mean PM2.5 exposures ranged from 9.0 μg/m3 [41] to 21.3 μg/m3 [42] and for PM10 from 2.97 μg/m3 [43] to 89.7 μg/m3 [44].
Methodological Quality of Studies
We used Downs and Black checklist to assess the methodological quality of studies related to BW and PTB (Table S1). We evaluated 27 studies and all of them were cohort or case-control studies. We scored “0” for eight checklist items which were applicable to randomized controlled trials but not relevant to our study. The total scores ranged from 11 to 19 for all included studies, with a median score of 15. Fourteen studies were regarded as relatively high quality (score ≥ 15) and thirteen were ranked as relatively low quality (score < 15). Most high quality studies assessed potential confounding bias and provided a clear explanation on the inclusion and exclusion criteria as well as follow-up procedures.
Quantitative Data Synthesis
The results of the pooled analyses are illustrated in Figures 2-4, forest plots showing effect estimate from individual studies for PM10. We reported PM10 as the number of effect estimate from studies was generally greater and results were more consistent than for the PM2.5. Forest plots for the PM2.5 are found in Figures S1-S5. The pooled ES of BW during pregnancy was -13.88 g (95% CI, -15.70 to -12.06 g) with a moderate heterogeneity among studies (I-squared=57.5%, p= 0.064) (Figure S1A). The pooled smoking-adjusted estimate was greatest for the entire pregnancy (ES, = -22.17 g; 95% CI, -37.99 to -6.41 g; I-squared = 92.3%, p< 0.001) compared to individual trimesters (Table 2 and Figure S1B).
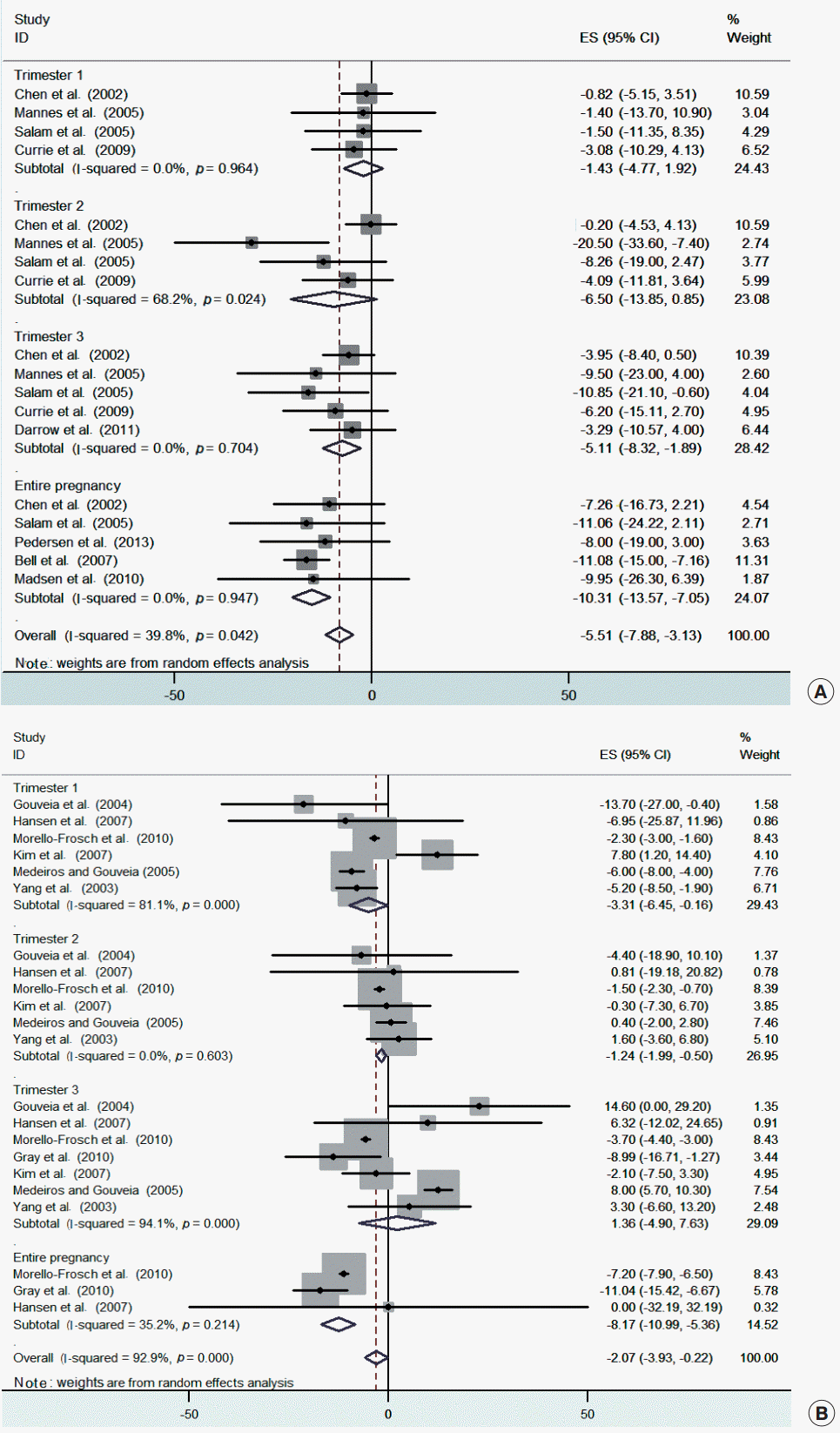
Effect size (ES) and 95% confidence interval (CI) of change in birth weight (g) per 10 μg/m3 PM10, by exposure period; size of shaded area around point estimate is proportional to weight in calculating pooled estimate. (A) Forest plot of pooled studies adjusting for smoking and (B) forest plot of pooled studies not adjusting for smoking. PM10, particulate matter less than or equal to 10 μm in diameter.

Effect size (ES) (odds ratio) and 95% confidence intervals (CI) for preterm birth per 10 μg/m3 PM10, by exposure period. PM10, particulate matter less than or equal to 10 μm in diameter.
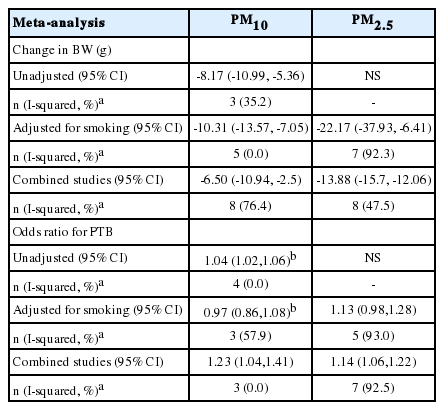
Summary of pooled estimates of effect for change in birth weight (BW) and preterm birth (PTB) in association with a 10 μg/m3 increase in maternal exposure to PM10 and PM2.5
Sixteen studies were included in the primary meta-analysis for the change in BW associated with a 10 μg/m3 increment in PM10, by gestational period. We found the pooled smoking-adjusted ES during pregnancy (ES, -10.31 g; 95% CI, -13.57 to -7.05 g; I-squared = 0.0%, p= 0.947) (Figure 2A) was larger than that without adjustment for smoking (ES, -8.17 g; 95% CI, -10.99 to -5.36 g; I-squared = 35.2%, p= 0.214) (Figure 2B). Pooled ES were generally of greatest magnitude based on exposure over the whole pregnancy (Figure 2). Combination of the eight better quality studies demonstrated a pooled overall ES of -6.07 (95% CI, -8.61 to -3.54) with higher heterogeneity (I-squared = 53.8%, p= 0.002) (Figure 3A); the pooled ES for eight studies with relatively low quality was -1.03 g (95% CI, -2.90 to 0.84 g; I-squared = 93.5%, p< 0.001) (Figure 3B). Similarly, compared to the low quality studies, the pooled estimate of relatively high quality was greatest for the entire pregnancy (pooled ES, -10.59 g; 95% CI, -13.24 to -7.94 g) with no heterogeneity (I-squared = 0%, p= 0.939).
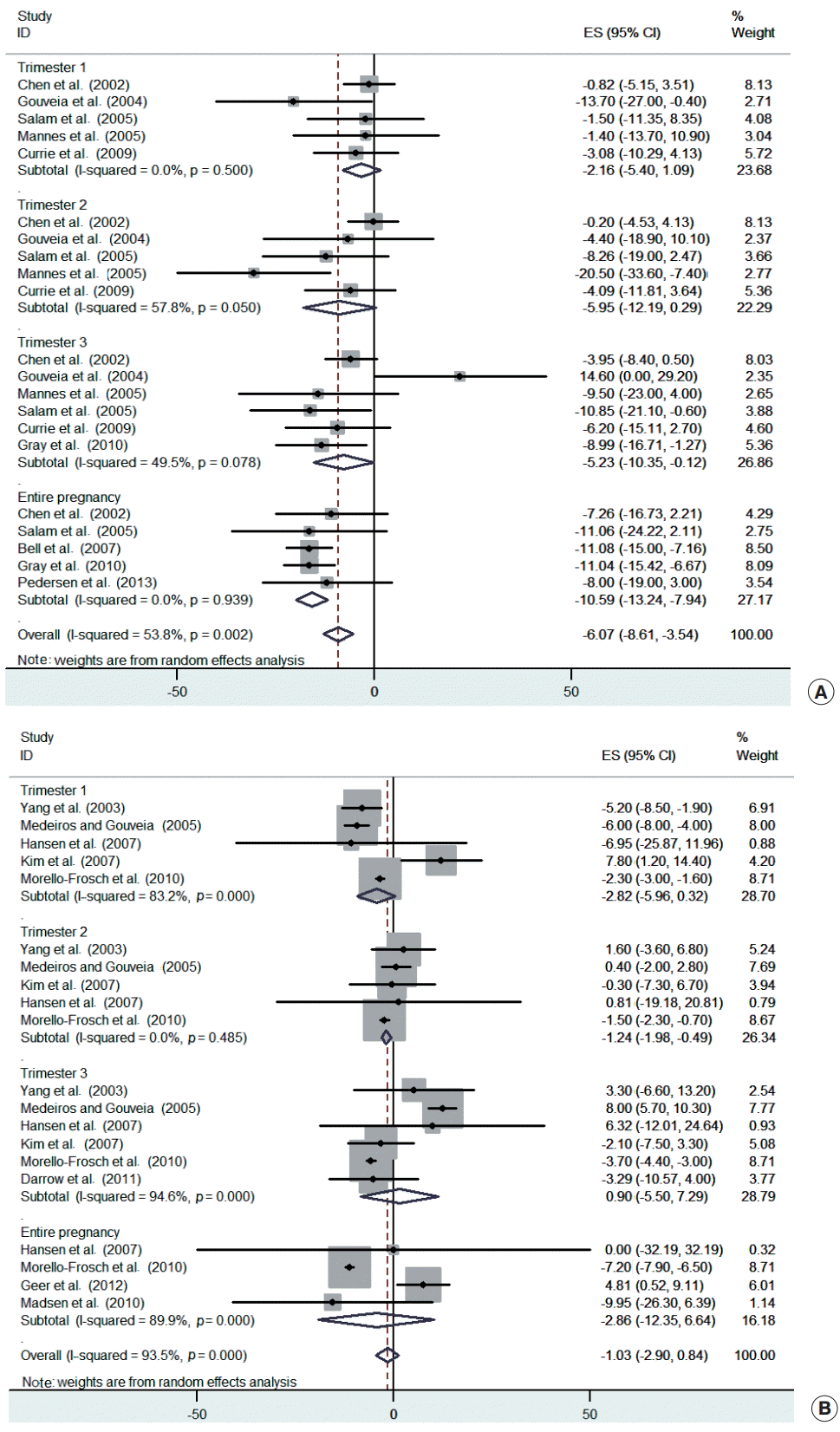
Effect size (ES) and 95% confidence interval (CI) of change in birth weight (g) per 10 μg/m3 PM10, by exposure period; size of shaded area around point estimate is proportional to weight in calculating pooled estimate. (A) Forest plot of pooled relatively better quality studies and (B) forest plot of pooled relatively low quality studies. PM10, particulate matter less than or equal to 10 μm in diameter.
A forest plot for PTB associated with a 10 μg/m3 increment in PM10 is presented in Figure 4. We estimated decreased odds of PTB for the first (combined OR, 0.98; 95% CI, 0.94 to 1.03) and second trimesters (combined OR, 0.97; 95% CI, 0.95 to 0.99), and increased odds for the third trimester (combined OR, 1.03; 95% CI, 1.01 to 1.05) and the entire pregnancy (combined OR, 1.23; 95% CI, 1.04 to 1.41). Heterogeneity among estimates was low, except for the first trimester, where it was high. Combination of studies that were based on methodological quality and confounding effect of smoking for PTB indicated mixed associations (Figures S3 and S4). We found the pooled smoking-adjusted OR (0.97; 95% CI, 0.86 to 1.08; I-squared = 57.9%, p= 0.093) was smaller than that without adjustment for smoking (pooled OR, 1.04; 95% CI 1.02 to 1.06; I-squared = 0.0%, p= 0.449) for the third trimester of exposures (Table 2), suggesting that a residual confounding of around 6.73% (1-0.97/1.04) was likely from smoking. Furthermore, we analyzed the studies by using the combination of third trimester or entire pregnancy into single group and stratified by smoking adjusted vs. smoking unadjusted categories, revealing the pooled OR of 1.03 (95% CI, 1.01 to 1.05) for smoking-unadjusted studies and 1.01 (95% CI, 0.90 to 1.13) for smoking-adjusted studies (Figures S5), indicating a residual confounding of around 2%. However, in other gestational periods, we could not compare the confounding effects of smoking due to lack of sufficient number of studies. Furthermore, we estimated that each 10 μg/m3 increase in PM2.5 exposure during pregnancy increase the risk of PTB by 14% (95% CI, 1.06 to 1.22) (Table 2).
Sensitivity Analyses and Publication Bias
With some noted exception, overall, we observed that meta-analysis estimates were stable, excluding a particular study did not change the summary point estimates much. For example, the pooled estimated reduction in smoking adjusted BW during pregnancy with a 10 μg/m3 increase in PM10 increased from -6.46 g (95% CI, -14.20 to 1.28 g) to -10.31 g (95% CI, -13.57 to -7.05 g) after removing the study by Geer et al. [15]. Likewise, removing the study by Medeiros and Gouveia [57] in smoking unadjusted BW during the third trimester was resulted in negative association with BW, changing ES from 1.36 g (95% CI, -4.90 to 7.63) to -1.63 g (95% CI, -5.84 to 2.48 g). For PTB and PM10 exposure, the meta-analyses were robust to the exclusion of influential studies [38,49,54] with regard to the magnitude of the estimated associations.
We did not detect a statistically significant publication bias based on the Egger’s test (p= 0.181 for PM10; p= 0.241 for PM2.5) or by using contour-enhanced funnel plot (Figure 5). The funnel plot revealed that studies were missing in areas of higher statistical significance, suggesting that asymmetry may be more likely to be due to factors other than publication bias, such as variable study quality [64].
Discussion
This systematic review has updated current scientific evidence and shows that decrease in BW per 10 μg/m3 increase in particulate matter (PM2.5 or PM10) during pregnancy; a decreased BW estimated with smoking-adjusted studies was consistently higher than the weight estimated with smoking unadjusted studies. We found the pooled estimates of decrease in BW of approximately 10 g for PM10 and 22 g for PM2.5 without evidence of publication bias or heterogeneity (PM10), after taking into consideration the confounding effect of smoking. In addition, our results of combined smoking-adjusted and-unadjusted studies suggest that maternal exposures to PM10 and PM2.5 during pregnancy are associated with 23% and 14%, respectively, excess risk of PTB.
We identified 13 previous reviews linking air pollution, BW and PTB [1-3,16-19,65-70]. Of the previous reviews, only two reviews have provided pooled estimates of effects [1,3]. Other previous reviews were basically provided a variety of qualitative observations and discussed outstanding methodological issues [3]. The major methodological issues identified included confounding factors, a small number of studies identified for each outcome, difference in characterization of exposure and outcome, and publication bias, although it was not quantified. There was little evidence to support the identification of a critical period of exposures. In a recent coordinated international analysis, Dadvand et al. [70] explored the influence of site characteristics and exposure assessment methods on between-center heterogeneity to quantify the association between maternal exposure to PM and term BW and low BW. However, this study was mainly based on center-specific effect estimates and maternal smoking was not included in their meta-regression analysis. One advantage of this review is that we appraised all individual studies included in the outcome specific analysis according to a structured and validated checklist, helping us to present quality assessment of methodological rigor of studies in a more organized and standardized way. The included studies allowed us to explore possible exposure-response relationship according to a critical exposure period, which offers another advantage of this meta-analysis.
Our pooled estimates of decrease in BW of 10 g and 22 g for each 10 μg/m3 increase in PM10 and PM2.5, respectively, is comparable with a recent coordinated international analysis [70] and a previous review [3]. A coordinated international analysis from 14 centers in 9 countries reported that PM10 was associated with an 8.9 g (95% CI, -13.2 to -4.6 g) decrease in BW per 10 μg/m3 in the fully adjusted random effects analysis, and there was significant heterogeneity among site characteristics. Stieb et al. [3] estimated decreases of 23.4 g (95% CI, -45.5 to -1.4 g) BW per 10 μg/m3 increases in PM2.5. As reported in previous reviews, we also observed a significant heterogeneity among the studies, but the degree of heterogeneity was varied considerably according to pollutants, outcome, and exposure period. However, we detected a consistent pattern of variability in estimates by gestational period.
We found overall or pooled smoking-adjusted ES for BW was stronger than that without adjustment for smoking in most cases. Combined smoking-unadjusted ES indicated a decrease in BW associated with a 10 μg/m3 increase in PM10 exposure during pregnancy (-8.17 g; 95% CI, -10.99 to -5.36 g). The association was stronger when adjusted for smoking (-10.31 g; 95% CI, -13.57 to -7.05 g). The strength and direction of this association was comparable with a previous fully adjusted (vs. unadjusted) random effects meta-analyses for term BW (-8.9 g; 95% CI, -13.2 to -4.6 g) [70].
We found that the pooled OR of PTB during entire pregnancy is higher than that of previous review. Sapkota et al. [1] estimated the association between PTB and maternal PM10 exposure during the third trimester (5 studies) and entire pregnancy (7 studies), but did not detect association with exposure in the entire pregnancy (OR, 1.02; 95% CI, 1.01 to 1.03 and OR, 1.02; 95% CI, 1.00 to 1.03, respectively). Our estimate for third trimester (OR, 1.03; 95% CI, 1.01 to 1.05) is in agreement with that study. We estimated a 23% increase in risk of PTB for each 10 μg/m3 increase in PM10 during entire pregnancy without evidence of heterogeneity (I-squared = 0%, p= 0.977). This may be due to fewer estimates available in that gestational period. One more possibility is that very high rates of PTB observed in some of the studies may have partly affected the pooled estimates. Stieb et al. [3] also reported higher risk of PTB for each 20 μg/m3 increase in PM10 during entire pregnancy (OR, 1.35; 95% CI, 0.97 to 1.90), but their lower 95% CI included the null value of 1.
We found no evidence of publication bias based on contourenhanced funnel plot for PM and BW. Therefore, we expect that any publication bias, if present, is minimal in our review. Thus, some difference in the ESs between this meta-analysis and previous one may be due to publication bias. A previous study that demonstrated a comparison between meta-analyses and large multicenter analyses of the associations between air pollution and mortality has suggested that difference in ESs reported in meta-analyses could be attributable to publication bias [71].
Woodruff et al. [14] stated that methodological quality of studies on air pollution and birth outcomes depends on alternative approaches to address residual confounding by individual risk factors, characterization of exposure patterns and confounding, refined characterization of critical exposure windows, evaluation of air pollution as a multi-pollutant mixture, and use of alternative pollutant metrics. We observed significant heterogeneity for some exposure periods. Variations in population characteristics, methods of exposure ascertainment, and characterization of confounding may have resulted in heterogeneity in the pooled estimates. Subgroup analyses in our review identified potential confounding effects of smoking and methodological quality (high or low) as potential sources of heterogeneity. We observed some attenuation in PM10 effect estimates for PTB after controlling for smoking, although the level of effect was less than 7%, suggesting that a residual confounding by unreported smoking is likely. However, we judged that, for PTB, there were too few estimates in individual exposure period in order to examine sources of heterogeneity in each period.
Although we realized that the countries where studies were conducted and the study design might also be sources of heterogeneity, they were not analyzed in the review due to the limited number of studies conducted in different countries. Though we recognized that several sensitivity analyses were conducted in relation to race or other factors, stratified analyses were not performed based on these categories due to the limited number of studies, particularly when divided by exposure period. We also aware that the use of effect estimates based on associations with ambient levels of pollutants as a surrogate for personal exposure levels may have resulted some exposure misclassification. Other limitation includes the fact that none of the included studies provided the precise information on the timing of smoking during pregnancy. Hence, future large cohort studies with sufficient data and detailed information on timing of smoking during pregnancy and other potential confounding factors as well as reliable exposure data are required for a better understanding of the association between PM and the risk of adverse birth outcomes.
Conclusion
This systematic review and meta-analysis, which are based on 44 studies of particulate pollution and birth outcomes, revealed that 10 to 22 g decrease in BW was linked to maternal exposure to particulate pollution (PM10 or PM2.5) during pregnancy, after adjustment for smoking. Likewise, pooled smoking-adjusted OR for PTB (0.97; 95% CI, 0.86 to 1.08; I-squared = 57.9%, p= 0.093) was smaller than that without adjustment for smoking (pooled OR,1.04; 95% CI, 1.02 to 1.06; I-squared = 0.0%, p= 0.449) for the third trimester of exposures, suggesting some attenuation in the pollution parameter after controlling for smoking. We identified potential confounding effects of smoking and methodological quality as potential sources of heterogeneity. There was considerable variability in pooled estimates of effect by gestational period. Our findings have substantial public health implications as reduced BW, although relatively small, is a risk factor for numerous adverse health effects early in life. In addition, it has also been associated with multiple adverse outcomes (reduced stature, increased incidence of cardiovascular disease, type 2 diabetes mellitus, and osteoporosis) later in life. Considering the ubiquitous nature of particulate air pollution [72]. exposure, variation in effects by exposure period, especially time periods shorter than trimester and sources of heterogeneity between studies and centers should be further explored.
Acknowledgements
The authors would like to thank Professor Nick Black and Eun Hee Ha for their helpful response to our queries. This study was funded by Inha University and Korea Centers for Disease Control and Prevention (#2014ER270501).
Notes
The authors have no conflicts of interest associated with material presented in this paper.
Supplementary Material
Quality assessment of individual studies based on Downs and Black checklist
Effect size (ES) and 95% confidence interval (CI) of change in birth weight (g) per 10 μg/m3 PM2.5, by exposure period; size of shaded area around point estimate is proportional to weight in calculating pooled estimate. (A) Forest plot of pooled studies and (B) forest plot of pooled studies adjusting for smoking. PM2.5, particulate matter less than or equal to 2.5 μm in diameter.
Effect size (ES) (odds ratio) and 95% confidence intervals (CI) for preterm birth per 10 μg/m3 PM2.5, by exposure period. (A) Forest plot of pooled studies and (B) forest plot of pooled studies adjusting for smoking. PM2.5, particulate matter less than or equal to 2.5 μm in diameter.
Effect size (ES) (odds ratio) and 95% confidence intervals (CIs) for preterm birth per 10 μg/m3 PM10, by exposure period. (A) Forest plot of pooled studies adjusted for smoking and (B) forest plot of pooled studies not adjusting for smoking. PM10, particulate matter less than or equal to 10 μm in diameter.
Effect size (ES) (odds ratio) and 95% confidence interval (CI) of preterm birth per 10 μg/m3 PM10, by exposure period; size of shaded area around point estimate is proportional to weight in calculating pooled estimate. (A) Forest plot of pooled relatively better quality studies and (B) forest plot of pooled relatively low quality studies. PM10, particulate matter less than or equal to 10 μm in diameter.
Effect size (odds ratio) and 95% confidence interval (CI) of preterm birth per 10 μg/m3 PM10 exposure during third trimester or entire pregnancy and stratified by smoking status. PM10, particulate matter less than or equal to 10 μm in diameter.